Lasers for Cold Atoms and Molecules
Cooling atoms to ultralow temperatures has produced a wealth of opportunities in fundamental physics, precision metrology, and quantum science.
Laser cooling of molecular gases is challenging to implement owing to the complexity of molecular structures. Recent application of sophisticated cooling techniques to molecules has opened the door to the longstanding goal of precisely controlling molecular internal and external degrees of freedom and the resulting interaction processes. This line of research can leverage fundamental insights into how molecules interact and evolve to enable the control of reaction chemistry and the design and realization of a range of advanced quantum materials. Cold molecule gases also allow precision measurements which set a new limit for electron’s electric dipole moment and could rule out many beyond standard-model physics theories.
C-WAVE at work with trapped ions
In this applications note we read how the flexibility of the C-WAVE’s single frequency tunable wavelength can be utilized for ion trapping experiments.
Coulomb crystals consisting of isotopically pure Magnesium ions are build employing a new tunable continuous-wave (cw) laser light source: Mg atoms are isotope-selective ionized by resonant two-photon excitation at a wavelength of 285.3 nm. The UV laser light is generated via resonant second-harmonic generation of the output of a new cw laser C-WAVE that offers about 0.5 W single frequency output power that is tunable in the range 450 – 650 nm. The created Mg ions are trapped and cooled, building 2D Coulomb crystals which are used for further investigation.
Single frequency, ultra low noise lasers for atom trapping
Fiber lasers are frequently employed for trapping of cold atoms due to their high output powers, ultra-low noise level, single-frequency emission, outstanding pointing stability, nearly perfect Gaussian beam profile, and best-in-class power stability (typically < ± 0.3% in the short and < ± 0.5% in the long term).
Related products
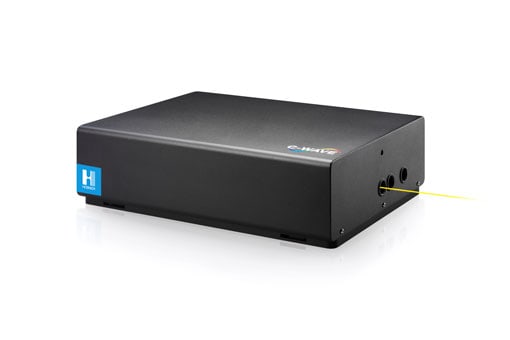
C-WAVE Series
Widely tunable continuous-wave lasers
Wavelength: 450 nm – 1900 nm
Power: 200 mW – 1000 mW
Applications: Nanophotonics, quantum research, Raman (TERS)