Lasers for Raman Spectroscopy
Raman spectroscopy basics
The ”inelastic scattering of light”, or Raman effect, was observed in practice for the first time already in 1928 by C.V. Raman for which he was awarded the Nobel Prize in 1930. It is only in the last two decades, however, that Raman spectroscopy has begun to realize its potential as an almost universally applicable analytical technique, not only in material and life science research applications, but also as a process control tool in, for instance, pharmaceutical, food & beverage, chemical and agricultural industries.
A number of technology advancements in recent years have aided instrument manufacturers in overcoming the challenge of weak signals associated with Raman spectroscopy and enabled development of small, sensitive and user-friendly Raman instruments. These advancements include improvements in laser technology; smaller, more powerful lasers with more robust spectral performance at many different wavelengths, detectors; CCDs and InGaAs arrays that allow designs of instruments without moving parts, spectral filters; Volume Bragg Grating elements for more narrow notch filters, along with developments of new schemes for Raman signal generation and detection.
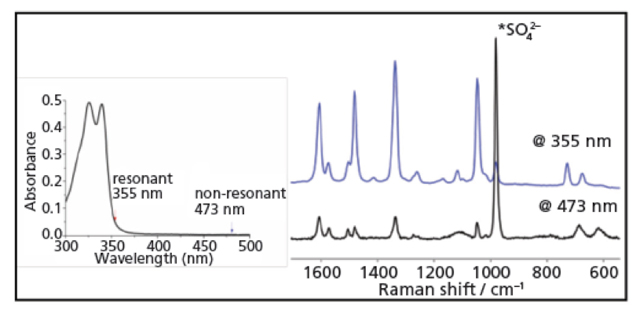
Fundamentally, Raman spectroscopy relies on illuminating a sample with a laser beam and detecting small spectral shifts in the scattered light that is caused by the interaction of the laser light with the chemical contents of the samples. Raman spectroscopy instruments come in wide range of different configurations; there are fiber-probe based Raman systems for in-line process control, ultra-high resolution Raman microscopes capable of imaging biological samples even at single-molecular level and more lately also very compact hand-held Raman devices for in-field inspection applications, e.g for detection of explosives or forensic investigations.
In pharmaceutical industries for instance, Raman-based tools are implemented throughout the whole manufacturing chain; for new drug development in biomedical laboratories through high through-put screening techniques, for quality inspection of raw materials for pharma production, for monitoring of granulation, blending and drying during pharma production and finally for non-invasive testing of produced tablets and gelcaps using high resolution chemical imaging.
Topographic Raman image of a tablet showing the distribution of the tables compounds along the surface (co. of Witec).
Which laser wavelength works best?
A number of different wavelengths are commonly used in Raman Spectroscopy today, ranging from the UV, over the visible, and into the near IR (NIR). Choosing the best illumination wavelength for a given application is not always obvious. Many system variables must be considered in order to optimize a Raman Spectroscopy experiment, and several of them are connected to the wavelength selection. Shorter wavelength gives (much) stronger Raman signal than longer wavelengths and shorter wavelengths allows use of cost-efficient, fast and low-noise CCD detectors (up to ~800 nm). Shorter wavelengths also enables higher resolution in imaging. But shorter wavelengths on the other hand usually results in much higher background noise from fluorescence from the samples. With these considerations taken into account it turns out that for many applications the best wavelength is around 780-790 nm. However, for highly fluorescent materials it might be needed to go to longer wavelengths in the NIR. Illumination in the visible is also becoming more and more popular, especially for inorganic materials and for use with resonance Raman and Surface-Enhanced Raman Spectroscopy (SERS).
Furthermore, in a growing number of cases it is beneficial to go to even shorter wavelengths, into the UV. Here the Raman signal is much stronger, and at short wavelengths there are some possibilities to filter out the Raman signal from the fluorescence background. Finally, in order to utilize resonance effects to strongly enhance the Raman signal it is in some cases preferred to have a broadly tunable narrow-linewidth illumination source. Understand how all the laser specifications influence the selection of lasers to be used for Raman spectroscopy in our post “How to choose lasers for Raman spectroscopy“.
The Cobolt lasers offer the world’s broadest range of compact lasers perfectly suited for high resolution Raman spectroscopy applications. Stable single-frequency operation combined with the ultra-robust thermo-mechanical architecture of HTCure provides narrow linewidths (<1 MHz for all DPLs) over a large range of operating temperatures, extremely low spectral drift (<1 pm) and a spectral purity guaranteed better than 80 dB (typically much better), which allows for very high resolution Raman spectroscopy and a possibility to detect low frequency Raman signals even down in the THz regime.
The tunablility of the C-WAVE in combination with the single frequency output make the C-WAVE also very well suited to high resolution Raman spectroscopy at resonance wavelengths, in particular in niche techniques such as tip enhanced Raman spectroscopy (TERS).
Want to learn more on how to select the right lasers, see our latest webinar:
How to select a laser for Raman Spectroscopy
Hosted by:
Or learn about our 08-NLDM 785 nm laser with enhanced spectral purity in our Laser Lounge Series of webinars:
Related products
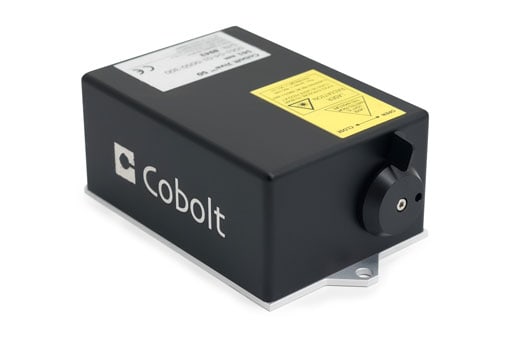
Cobolt 04-01 Series
Single frequency, CW diode pumped lasers
Wavelength: 457 nm – 1064 nm
Power: 25 mW – 400 mW
Applications: Raman, microscopy, LDV, DLS
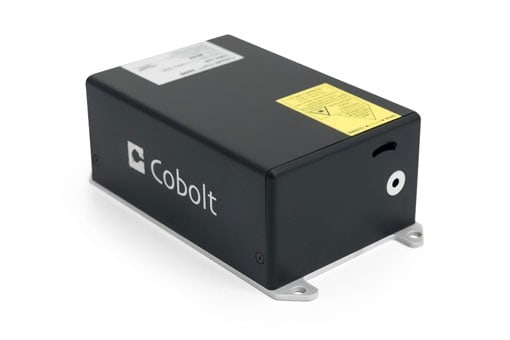
Cobolt 05-01 Series
High power, single frequency, CW diode pumped lasers
Wavelength: 320 nm – 1064 nm
Power: 10 mW – 3000 mW
Applications: Holography, Raman, microscopy, flow cytometry, research
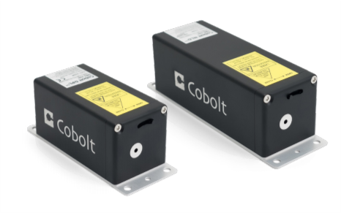
Cobolt 08-01 Series
Compact narrow linewidth lasers
Wavelength: 405 nm – 1064 nm
Power: 25 mW – 500 mW
Applications: Raman spectroscopy, interferometry, dynamic light scattering.
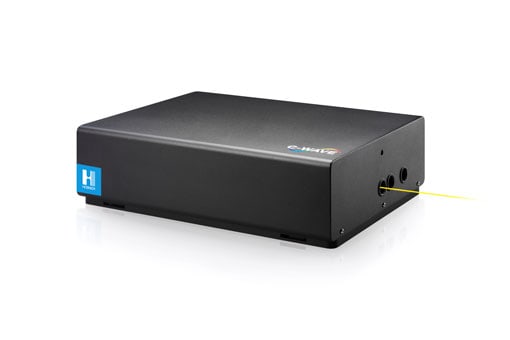
C-WAVE Series
Widely tunable continuous-wave lasers
Wavelength: 450 nm – 1900 nm
Power: 200 mW – 1000 mW
Applications: Nanophotonics, quantum research, Raman (TERS)
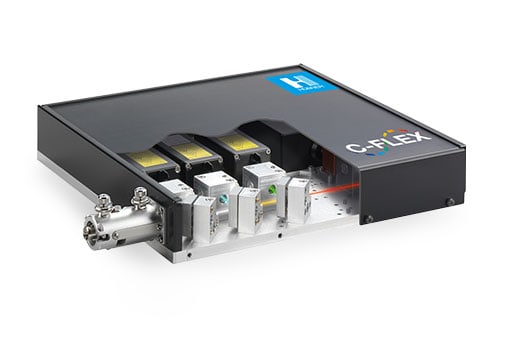
C-FLEX
The compact and flexible laser combiner
Wavelength: 375 nm – 1064 nm
Power: 50 mW – 1000 mW
Applications: Microscopy, Raman, holography
Publication N Mueller et al Tip enhanced Raman spectroscopy JPhysChem C 2018
Editorial Low frequency Raman Spectroscopy Physics Best 2020
Editorial Tunable lasers for tip enhanced Raman Physics Best 2019
Editorial Novel tunable lasers enable Tip Enhanced Raman LFW 2019
Editorial How to choose a laser for Raman Spectroscopy, LFW 2018
Apps note Lasers for low frequency Raman spectroscopy
Apps note Raman and light sheet microscopy
Apps note Lasers for Raman using 785 nm
Apps note Lasers for Raman using 405 nm narrow linewidth
Apps note Imaging of Ultrathin Graphite with Confocal Raman Imaging at 355nm Imaging & Microscopy
Apps note High Speed 785 nm NIR lasers for Raman Spectroscopy
Apps note Have I selected the right laser for Raman
Apps note High performance lasers for Raman spectroscopy
Apps note Cobolt Mambo™ 594 nm for Raman spectroscopy
G.Strömqvist et. al. Suppression of Raman scattering in PPKTP. ECLEO 2009